電動モーターの効率を引き出す: ヘアピンストリッピングの力
Authors: Authors: Jessica Schober, Dmitry Badyukov, and Malte Hemmerich on Unlocking Efficiency In Electric Motors: The Power Of Hairpin Stripping
要約
This paper presents an in-depth analysis of the hairpin stripping process for efficient stator production in electric motors and generators. The study focuses on optimizing laser parameters and equipment setup, with particular attention to the integration of a dual wavelength 3-axis scanning head that helps to reduce complexity and machine size. This advanced scanning technology enhances the precision and efficiency of the stripping process. Application examples demonstrate the effectiveness of the approach, highlighting improved outcomes in electric motor and generator manufacturing.
Hairpin Stripping Process
In the production of stators for electric motors and generators, hairpins play a vital role. Hairpins are rectangular copper wires coated with an organic insulation layer, offering advantages such as improved copper fill factor and enhanced motor efficiency compared to round and flexible insulated copper wires. However, the thicker profile of hairpins poses challenges in terms of traditional winding techniques and mechanical removal of the insulation material. Additionally, a substantial number of welds are required to connect the hairpins after the assembly of the stator.
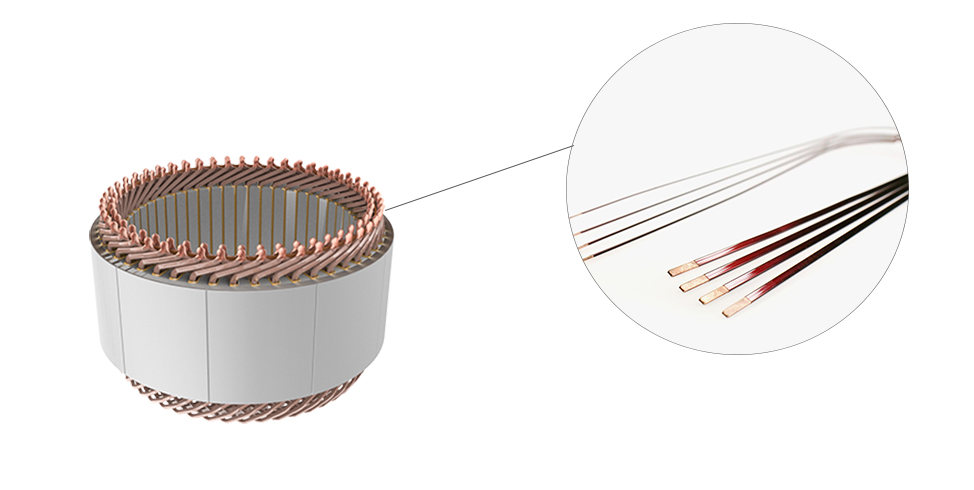
To ensure efficient and high-quality welding of hairpin ends, it is crucial to have a clean and residue-free copper surface. The cleanliness of the hairpin surfaces directly impacts the quality of the welds and the overall performance of the motor. Therefore, a critical process known as hairpin stripping is used during hairpin production to remove the insulation or enamel from the rectangular copper profiles.
Recognizing the importance of efficient and precise hairpin stripping, Novanta has been approached by industry leaders with specific application requests. These requests typically involve stripping approximately 30mm sections on all four sides of rectangular copper profiles measuring around 3mm x 1.5mm. Moreover, the hairpin stripping process needs to be completed within a short cycle time, typically less than 1.5 seconds.
Materials and Wavelengths
To determine the best approach for the hairpin stripping process, a careful analysis of the materials and their absorption spectrum for available industrial laser wavelengths is essential. Commonly used insulations in hairpins include organic composites such as Polyamide (PA), Polyether (PE), and Polyimide (PI), with pure copper as the base material. The goal of the process is to effectively remove the entire organic layer while leaving the base material unmodified.
Organic materials show strong absorption within the far infrared (FIR) spectrum. However, since specific compounds may deviate from standard absorption data, we employ an advanced 2.5µm-25µm ATR (attenuated total reflection) spectrometer in our laboratory. This instrument allows us to analyze the absorption behavior of organic materials in the IR-FIR wavelength regime and determine the ideal wavelengths for further testing.
Through our analysis, we have confirmed that the organic insulation layers exhibit significant absorption at longer wavelengths within the FIR spectrum. Based on this understanding, we have chosen CO2 lasers as the best solution for insulation removal from the copper wires. Our Synrad CO2 lasers operate at specific wavelengths of 9.3µm, 10.2µm, and 10.6µm, offering a versatile range of spectra that align with the absorption curves of different insulation materials. Furthermore, these lasers show high reflectivity on the pure copper base, ensuring efficient insulation removal while minimizing the risk of discoloration or surface modification.
Although the CO2 laser effectively removes most of the insulation material, a thin residue measuring 1-2µm in thickness may remain on the reflective copper surface. This residual layer cannot be effectively eliminated by FIR radiation alone. Consequently, a second process step utilizing a low-power nanosecond fiber laser is employed to ensure complete removal of this residue. This fiber laser possesses better absorption characteristics in the base material, enabling thorough stripping while safeguarding the copper surface from excessive heat and surface modification.
While alternative methods such as laser ablation using short-pulsed lasers or a single, more powerful fiber laser exist for insulation removal, the two-step process we employ offers several advantages in terms of effectiveness, quality, and processing speed. True ablation processes often lack the necessary speed and do not differentiate between the insulation material and the base material. On the other hand, using a single, more powerful fiber laser introduces a higher level of heat and surface modification to the base material. Moreover, both alternatives require significant investments in laser sources. Therefore, the two-step process remains the ideal solution for the hairpin stripping process, striking a good balance between efficiency, quality, and cost-effectiveness.
Equipment for the Hairpin Stripping Process
To execute the two-step hairpin stripping process described earlier, the setup requires a minimum of two lasers and two galvanometer-based scanning heads. However, as the process involves treating all four sides of the hairpin, the equipment requirements, costs, and complexity increase significantly. Additionally, the inclusion of two cycle positions within the manufacturing process results in larger machine size.
Our tests indicate that the initial step of the process can be efficiently performed using a 400W continuous-wave CO2 laser operating at 10.6µm, such as the Novanta Firestar i401. Focusing the laser to a moderate spot size of >300µm yields excellent results. For the second step, a 200W single-mode nanosecond laser with a smaller focal spot is necessary. Optimal cleaning of two sides per step can be achieved by positioning the hairpin beneath a 3-axis scanning head at a 45° angle.
Based on these findings and requirements, we have developed a subsystem that minimizes overall equipment complexity and size. The Novanta Chameleon scan head has a uniquely designed housing that accommodates two 3-axis galvanometer scanners, utilizing a primary and secondary controller architecture. This independent galvo scanner can be customized for different wavelengths and focal sizes, while maintaining exactly the same working distance. When combined with a Novanta ASC Controller, a Novanta Firestar i401 CO2 laser, and an industrial-grade 200W nanosecond single-mode fiber laser, this solution enables a 75% overlap of the working fields. Simultaneously, both wavelengths can be independently utilized for efficient processing.
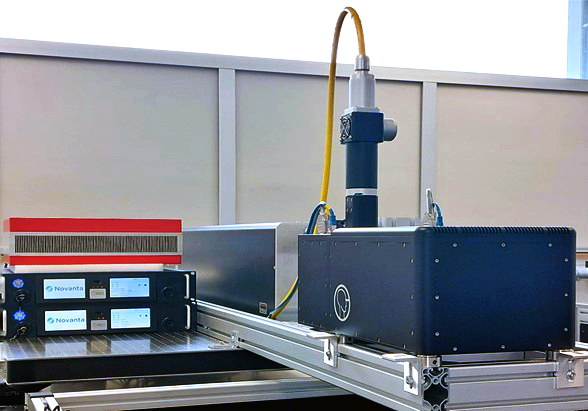
While high-quality equipment is crucial for process development, the ability to analyze and evaluate results after parameter changes is equally important. Establishing common methods between the application laboratory and end-users fosters effective collaboration. In the hairpin industry, fluorescence meters are commonly used to detect residual materials remaining on the surface after the process. This method effectively identifies organic materials, but its accuracy depends on factors such as measuring technique, position, and surface structure. Typically, these meters analyze ~1mm² at a time and provide relative fluorescence units (RFU) as results. In the industry, a low single-digit RFU is accepted as indicative of excellent quality. In our laboratory, we adhere to these industry standards but complement them with confocal and laser scanning microscopes. These advanced tools provide deeper insights into the internal mechanisms of the process following parameter changes, enhancing our understanding and optimization of the hairpin stripping process.
Application Examples for Hairpin Stripping
In this white paper, we conducted an analysis of four different hairpin designs before and after the laser cleaning process. These hairpins had varying insulation layer materials and thicknesses (see samples in Figure 3). Additionally, we measured the absorption of the four samples using the ATR IR-spectrometer. The results indicated an increased absorbance at a CO2 wavelength of 9.3µm for the insulation layer of all samples (see Figure 4). Consequently, we proceeded with the same laser setup for all hairpin samples.
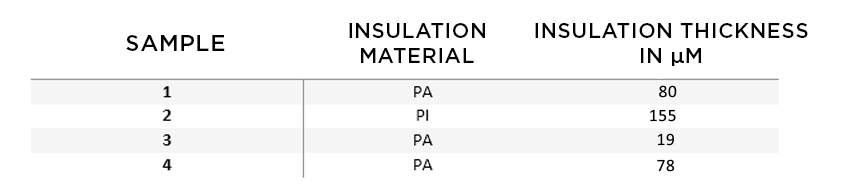
Figure 3 clearly demonstrates the variation in insulation thickness across a wide range. However, we found that the thickness had only a limited effect on the process parameters of the initial decoating step and no observable effect on the residual layer in that step.
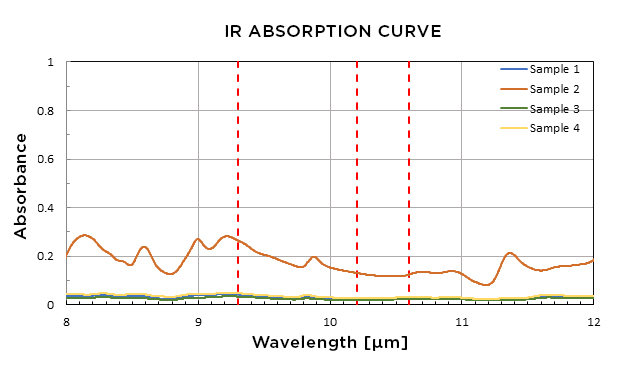
Figure 3 clearly demonstrates the variation in insulation thickness across a wide range. However, we found that the thickness had only a limited effect on the process parameters of the initial decoating step and no observable effect on the residual layer in that step.
For all the experiments described here, we used a combination of a 400W CO2 laser and a 200W nanosecond fiber laser. The working distance for both wavelengths was set to 360mm, resulting in a spot size of approximately 400µm for the CO2 laser and 40µm for the fiber laser. The overlapping field allowed both laser processes to run simultaneously. In these application examples, we ablated a 5×20 mm² area using a bi-directional raster scan.
The parameters for the CO2 and fiber processes were individually adjusted for each hairpin to achieve optimal cleaning results based on the different insulation materials, thicknesses, and hairpin diameters. Although the laser parameters differed, the total stripping process time remained consistent. All application samples could be fully ablated within a cycle time of less than 1.0s.
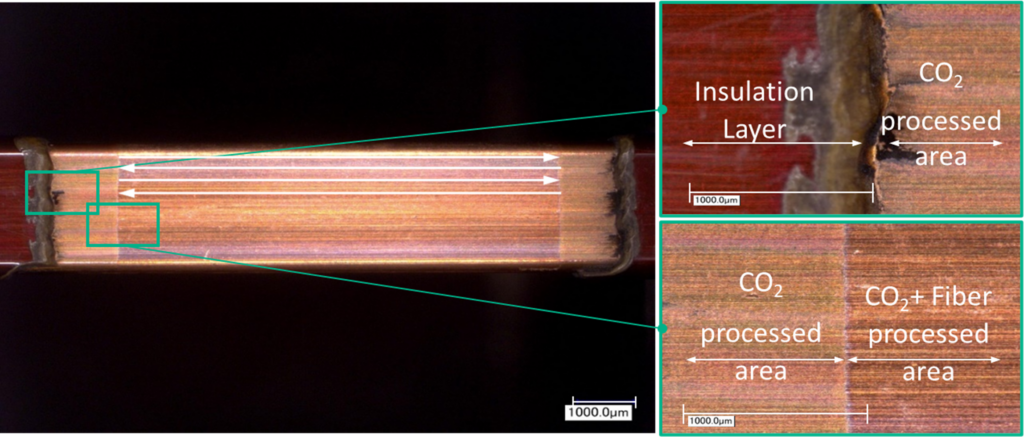
To visualize and analyze the individual process steps, we first removed the insulation layer using the CO2 laser over an increased area of 5×25 mm². Subsequently, the fiber laser process followed in the 5×20 mm² area. Figure 5 highlights the cleaned hairpin, with the left side representing the area where only the CO2 laser removed the insulation layer, and the right side showing the area where the fiber laser was used as the second process step.
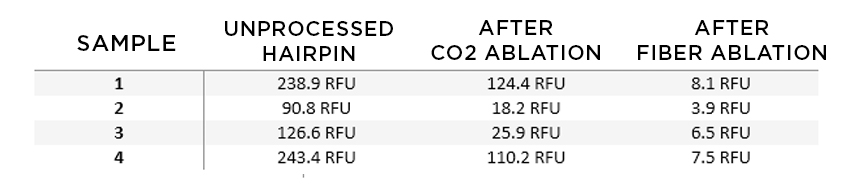
To measure the effectiveness of the laser processes, we performed fluorescence intensity measurements before and after each step. The hairpin samples were ablated as shown in Figure 5 to enable fluorescence intensity measurements. The area with the organic insulation layer showed the highest level of contamination. However, through laser processing, the contamination decreased, reaching a fluorescence intensity of less than 9 RFU after the fiber process for all samples (see Figure 6).
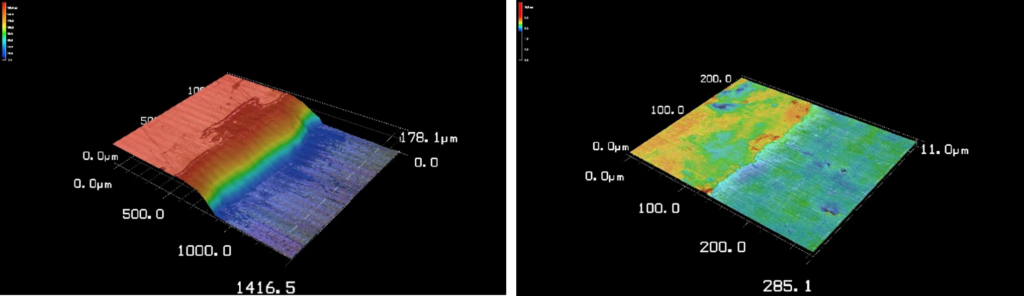
To analyze the amount of insulation layer removed, we employed a confocal laser scanning microscope. We measured the height of all application samples before and after the laser process. Figure 7 presents the measurement results of a representative sample in a three-dimensional view.
In Figure 8 it can be seen that the CO2 laser process removes all but about 1-2μm of the isolation layer regardless of its previous total thickness. However, employing the fiber laser as the second cleaning step allowed for the complete removal of the remaining 1μm thick insulation layer (right side of Figure 7).
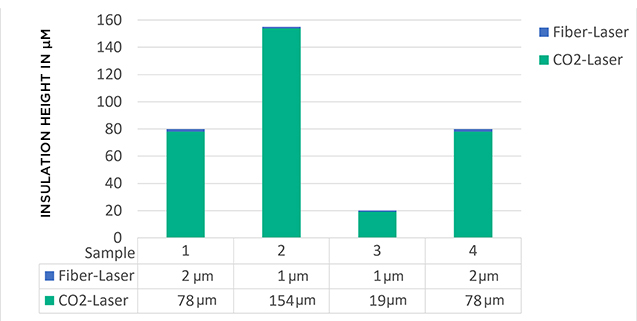
Figure 8 displays the amount of insulation removed using only the CO2 laser or the combination of both the CO2 and fiber laser sources.
With the initial CO2 laser process, more than 97% of the insulation material was removed, effectively cleaning most of the copper hairpin. The remaining 1-2µm thick insulation film could be eliminated completely using the fiber laser source. This second process step achieved insulation-free ablation, enabling subsequent welding on the hairpin ends.
結論
In this paper, we have presented a comprehensive analysis of the hairpin stripping process for efficient stator production in electric motors and generators. Our focus was on optimizing laser parameters and equipment setup, with a particular emphasis on the integration of a dual wavelength 3-axis scan head. This advanced scanning technology enhances the precision and efficiency of the hairpin stripping process.
By carefully analyzing the absorption behavior of organic materials commonly used as insulation in hairpins, we determined the optimal wavelengths for the laser sources. CO2 lasers operating at specific wavelengths of 9.3µm, 10.2µm, and 10.6µm were found to effectively remove the organic layer while minimizing the risk of surface modification. To eliminate the thin residual layer remaining on the copper surface, a low-power nanosecond fiber laser was employed as the second process step. Our approach of employing a two-step hairpin stripping process offers several advantages, including improved effectiveness, quality, and processing speed compared to alternative methods. Furthermore, it strikes a balance between efficiency, quality, and cost-effectiveness.
In summary, our study highlights the significance of optimizing the hairpin stripping process for efficient stator production and how Novanta Application Laboratories can help machine integrators achieve best performance. The integration of a dual wavelength 3-axis scan head, combined with CO2 and fiber lasers, offers enhanced precision, efficiency, and quality in the stripping process. These advancements contribute to improved outcomes in electric motor and generator manufacturing.
Novanta ベネフィッツ
Novantaは、高精度レーザーシステムを使用して製造プロセスを進化させたいOEM、システムインテグレーター、エンドユーザーの最も複雑な課題さえ解決するために独自の立場にあります。 業界で最も有名なブランドのいくつかと国内のアプリケーションおよびサービスサポートを備えて、Novantaは信頼性の高い、正確で耐久性のあるコンポーネントおよびサブシステムを提供しています。
当社のアプリケーションテストラボは、OEM、システムインテグレータ、材料メーカー、プロセッサ、および自動機械のエンドユーザにアプリケーションおよび概念のテストを提供しています。 Novantaのアプリケーションエンジニアはレーザー加工の専門家であり、成功と効率的なレーザー加工を確実にするパラメータを理解しています。 有名なNovantaブランドのレーザーとビームステアリング機器を使用し、当社のアプリケーションエンジニアは、望ましい結果を得るための主要な製品パラメータと処理ノウハウを決定します。