The Benefits of Ultrashort Pulses in Two-Photon Microscopy
Ultrashort Laser Pulses Offers Many Benefits
要約
The use of ultrashort laser pulses for two-photon microscopy (TPM) offers many benefits including; deeper imaging, higher excitation efficiency, reduced photo damage, simultaneous excitation of multiple fluorophores, with the promotion of auto-fluorescence.
Introduction
Since it was first reported in the early 1990s, two-photon microscopy has become a standard imaging technique in the life sciences laboratory due to the benefits it brings over conventional confocal microscopy. The highly localized excitation of the sample allows for depth measurement and a reduction in sample exposure to damaging energy.
Over this period the basic design of the microscope system, especially the excitation source, has remained largely unchanged – an ultrafast laser with a repetition rate of 80-100 MHz and a pulse duration in the range of 100-150 fs. This ‘standardization’ of two-photon microscopy as an imaging technique has in part been driven by the improvements made to the reliability of the laser and the reduced need for routine cleaning, general maintenance and aligning. In the early days of using Ti:Sapphire mode-locked lasers, the daily process of cavity attention meant a delay before microscopy could begin, and the need for a researcher to have photonics as well as microscopy expertise.
The Benefits of Ultrashort Pulses
It has been shown in numerous papers, that the use of ultra-short (<10 fs) pulses has important benefits for two-photon microscopy. With short pulses, the instantaneous peak power for a given average output is much higher. This results in reduced scattering allowing deeper imaging, higher fluorescent efficiency (figure 1) and improved image contrast, all without increasing the photo-damage inflicted on the sample [1][2]. The broad spectral output, using the entire emission range of the Ti:Sapphire, allows for multiple dye excitation without the need for laser tuning [3] and evidence of the promotion of auto-fluorescence [4], an ability of great interest to the community looking at live cell imaging.
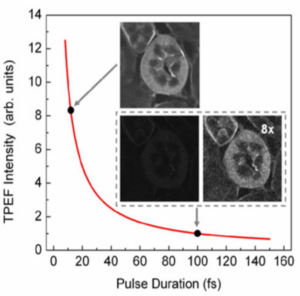
With all these recognized benefits, why have short pulses remained a specialist area? Perhaps one of the answers lies in the nature of the broadband pulses, which provide the majority of the benefits. The speed of light through a medium is dependent on its wavelength, and with a very wide wavelength range in a sub-10 fs pulse, the range of velocities becomes significant. Compounded by the complexity of the beam path in a microscopy, optics made from different media, all with different thicknesses, maintaining a short pulse at the sample becomes more of a challenge. Since the spectral make-up of a 150 fs pulse is very narrow, the difference in velocities is negligible.
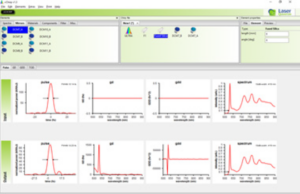
The principle method of ensuring the short pulse at the sample plane is to “pre-chirp” or provide an equivalent reverse delay to the pulse within the beam path. This can be done with a series of bounces off chirped mirrors, such as the DCM range, using glass wedges for fine tuning. Sounds simple, but how do you know how many bounces? The recently improved venteon vChirp software allows a user to input the various optics involved in the path of the beam between laser and sample, and will model the effect of negative dispersive elements to predict the best combination to ensure the short pulse at the point of need (figure 2).
In recent work, the ability to use the venteon ultra short pulse laser and the d-scan pulse characterization and compression unit has been reported [5]. The venteon ultra brings the capability of <5 fs transform-limited pulses with actual measured pulses of <5.5 fs, thus offering the benefits of enhanced excitation efficiency and direct excitation of multiple fluorophores due to the broad spectral output, as mentioned earlier in this article [3]. Here, the authors describe the use of the venteon ultra and the d-scan to compensate for the dispersion caused by a standard 63x, 0.75 numerical aperture objective.
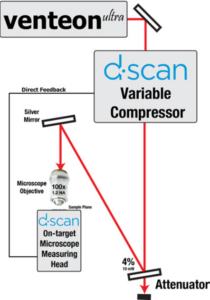
Being smaller, and having a direct feedback from the ‘point of use’ characterization unit to the compensation module, the d-scan was able to achieve a pulse after the objective of <5.5 fs. Figures 3 and 4 show the set-up and result. The benefits of ultra-short pulses are now available to the microscopy lab and the microscopist without the need for photonics expertise, resulting in huge advances to the life sciences industry as a whole.
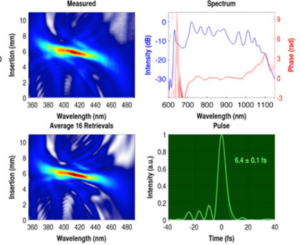
Novanta ベネフィッツ
Novantaは、高精度レーザーシステムを使用して製造プロセスを進化させたいOEM、システムインテグレーター、エンドユーザーの最も複雑な課題さえ解決するために独自の立場にあります。 業界で最も有名なブランドのいくつかと国内のアプリケーションおよびサービスサポートを備えて、Novantaは信頼性の高い、正確で耐久性のあるコンポーネントおよびサブシステムを提供しています。
当社のアプリケーションテストラボは、OEM、システムインテグレータ、材料メーカー、プロセッサ、および自動機械のエンドユーザにアプリケーションおよび概念のテストを提供しています。 Novantaのアプリケーションエンジニアはレーザー加工の専門家であり、成功と効率的なレーザー加工を確実にするパラメータを理解しています。 有名なNovantaブランドのレーザーとビームステアリング機器を使用し、当社のアプリケーションエンジニアは、望ましい結果を得るための主要な製品パラメータと処理ノウハウを決定します。
Acknowledgements
Andrew Wells, Laser Quantum
References:
[1] 1. Tang S et al, Effect of pulse duration on two-photon excited fluorescence and second harmonic generation in nonlinear optical microscopy, J Biomed Opt 11(2): 020501, March 2006. doi:10.1117/1.2177676
[2] Xi P et al, Two-photon imaging using adaptive phase compensated ultrashort laser pulses, J Biomed Opt 14(1): 014002, Jan-Feb 2009. doi: 10.1117/1.3059629
[3] Wang C et al, Two-photon excited fluorescence enhancement with broadband versus tuneable femtosecond laser pulse excitation, J Biomed Opt 17(2): 025003, February 2012
[4] Gibbs H et al, Imaging embryonic development with ultrashort pulse microscopy, Optical 53(5): 051506, May 2014
[5] Benefits of ultrashort pulses in two-photon microscopy. Visit: www. laserquantum.com